James Odell, OMD, ND, LAc
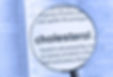
Cholesterol is a molecule critical to health. Unfortunately, it has been oversimplified and demonized by conventional medicine and the mainstream media as the major cause of heart disease and vascular endothelial dysfunction. This article (part 1 of 2) explores the bioregulatory importance of cholesterol and specific factors that can corrupt this molecule, leading to its potential participation in cardiovascular disease risk. Much of the reason for the keen interest in cholesterol has its roots in selling pharmaceuticals designed to lower cholesterol. That is a discussion too vast for this article and will be addressed in part 2 where I take a deep rabbit hole dive into the dark world of cholesterol-lowering medications (statins).
Bioregulatory Functions of Cholesterol
Cholesterol fulfills several biological functions and is necessary for successful cellular homeostasis. Every cell membrane in our body contains cholesterol because cholesterol is what makes our cells waterproof. Without cholesterol, we could not have a different biochemistry on the inside and the outside of the cell. When cholesterol levels are not adequate, the cell membrane becomes “leaky or porous”, a situation the body interprets as an emergency, releasing a flood of corticoid hormones that work by sequestering cholesterol from one part of the body and transporting it to areas where it is lacking. Thus, cholesterol is the body’s repair substance as we see scar tissue contains high levels of cholesterol, including scar tissue in the arteries.1, 2
As such, cholesterol helps in nerve conduction and is vital to proper neurological function. It plays a key role in the formation of memory and the uptake of hormones in the brain, including the all-important neurotransmitter serotonin. When cholesterol levels drop too low, the serotonin receptors cannot function. Cholesterol is a major component of the brain, much of it in the myelin sheaths that insulate nerve cells and in the synapses that transmit nerve impulses. This myelin sheath allows electrical impulses to transmit quickly and efficiently along the nerve cells. If myelin is damaged, these impulses slow down. The myelin sheath is rich in cholesterol because it is derived from compacted layers of the Schwann cell membrane. Schwann cells or neurolemmocytes are the principal glia of the peripheral nervous system which help in providing protection, and insulation, allowing for more efficient conduction of nerve impulses.3, 4
It helps in providing protection, and insulation and allows more efficient conduction of nerve impulses.3, 4
Cholesterol is an extremely important biological molecule that not only has roles in membrane structure and is important for neurological function, yet also acting as a precursor for the synthesis of steroid hormones. Cholesterol is the precursor to all the hormones produced in the adrenal cortex including glucocorticoids, which regulate blood sugar levels, and mineralocorticoids, which regulate the mineral balance and sex hormones. Corticoids are the cholesterol-based adrenal hormones that the body uses in response to the stress of various types; they promote healing and balance the tendency to inflammation. Cortisol allows the body to manage and respond to stress through its effects on intermediary metabolism, in other words, increased gluconeogenesis including inflammatory and immune responses. The adrenal cortex also produces sex hormones, including testosterone, estrogen, and progesterone, out of cholesterol. The hormones testosterone, estrogens, and progesterone are critical for reproductive bioregulation and responsible for sexual differentiation, libido, spermatogenesis, and ovarian follicle production.5, 6
Bile salts are composed of cholesterol and those who suffer from low cholesterol often have trouble digesting fats. It acts as a precursor to bile acids required for the digestion of fat and plays a central role in maintaining cellular membrane rigidity and fluidity. Bile is a watery mixture of both inorganic and organic compounds, of which phosphatidylcholine and conjugated bile salts/acids are quantitatively the most important. Between 15 and 30 grams of bile salts/acids are secreted from the liver each day, but as a result of bile reabsorption, only about 0.5 grams are lost daily in the feces. To replace the amount lost, roughly 0.5 grams per day is synthesized from cholesterol in the liver. Cholesterol is incorporated as the backbone in bile acid synthesis, a complex multistep, multi-organelle process. This synthesis creates a way for the body to excrete cholesterol as there is no way to break it down physiologically, and it allows lipids to be digested via emulsification and subsequent breakdown by pancreatic enzymes.7
Cholesterol is also important for the all-important production of vitamin D. Vitamin D3 (cholecalciferol), from either the skin or the diet, undergoes hydroxylation by 25-alpha hydroxylase to form 25-hydroxycholecalciferol (calcidiol) in the liver from lipid-soluble compounds with a 4-ringed cholesterol backbone. It is then further hydroxylated by 1-alpha hydroxylase to an active form of 1,25-dihydroxycholecalciferol (calcitriol) in the kidneys. Vitamin D plays an integral role in calcium homeostasis by mobilizing calcium from the bones and stimulating intestinal absorption and reabsorption in the kidneys. Vitamin D has other roles in the body, including the reduction of inflammation as well as modulation of such processes as cell growth, neuromuscular and immune function, and glucose metabolism Without adequate cholesterol, vitamin D remains deficient.
Thus, low cholesterol–whether due to an innate error of metabolism or induced by cholesterol-lowering drugs–can be expected to disrupt the production of adrenal hormones and lead to blood sugar problems, edema, mineral deficiencies, chronic inflammation, difficulty in healing, allergies, asthma, reduced libido, infertility, and various reproductive problems. Cholesterol is thought to function as an antioxidant and as such protects us against free radical damage that leads to heart disease and cancer. Low cholesterol levels are often associated with increased rates of cancer, as are low vitamin D levels.8, 9, 10
Oxidized LDL Cholesterol (ox-LDL) and LDL Particle Number (LDL-P)
It has been known for over two decades that the real problem is not simply elevated low-density lipoproteins (elevated LDL) but rather oxidized low-density lipoprotein (ox-LDL) cholesterol. Although very high levels of LDL cholesterol are generally not good for health (typically indicative of insulin resistance), the primary problem is not the LDL itself, but rather when it is oxidized. More than half (52%) of the people suffering a heart attack have “normal” cholesterol levels (at least “normal” before the standards were changed).
Cholesterol has a double bond that is susceptible to oxidation leading to the formation of oxysterols. An oxysterol is a derivative of cholesterol obtained by oxidation involving enzymes and/or pro-oxidants. Several oxysterols are associated with age-related diseases such as cardiovascular disease, eye disease (cataract, age-related macular degeneration), certain neurodegenerative diseases, and cancers.11, 12, 13, 14, 15
Low-density lipoproteins (LDLs), a major carrier of circulating cholesterol, are very susceptible to oxidation by pro-oxidants such as metal ions, reactive oxygen radicals, oxidized macrophages (white cells), lipoxygenase, and peroxynitrite. In particular, myeloperoxidase (MPO) oxidizes LDL, making it atherogenic. MPO is an enzyme released by white blood cells that are involved in disease activity from the luminal aspect of the arterial wall. When the artery wall is damaged or inflamed, MPO is released by white blood cells called macrophages. MPO then oxidizes LDL, making it atherogenic, which then oxidizes HDL (good cholesterol), rendering it dysfunctional. This results in inflammation linked to plaque formation in the artery wall, also known as vasculitis. MPO can be measured in the laboratory and it is seen that individuals with elevated MPO levels are more than 2x as likely to experience cardiovascular mortality.
When the LDL is oxidized, it becomes antigenic and the ox-LDL is taken up excessively by the “scavenger” or “ox-LDL receptors” on monocyte-derived macrophages. Ox-LDL is present in macrophages in atherosclerotic lesions but not in normal arteries. Once macrophages breach the arterial endothelial barrier, the excessive uptake of ox-LDL contributes to their entrapment in the subendothelial space. The trapped lipid-laden “foam” cells elicit biosynthesis and release of factors by the arterial wall that is proinflammatory, perpetuating the atherosclerotic process with injury to the arteries. Injury to the subendothelial vessel walls results in decreased production of nitric oxide and loss of elasticity of the arteries, and the damaged lipid-laden arteries eventually narrow, thereby restricting the flow of blood. Oxidized cholesterol not only directly damages the blood vessels, but it also serves as a marker of oxidative stress.16, 17
These oxidation by-products are formed from many foods during cooking or processing. They are cytotoxic, mutagenic, carcinogenic, and atherogenic. Eggs, milk, meats, and their products are the main dietary sources of oxysterols.18 Additionally, oxidative free radical pathology (oxidative stress) from infections, environmental toxins, and degenerative disease processes greatly participate in creating oxysterols.
Epidemiologic data suggest that decreased levels of micronutrient antioxidants are associated with an increased frequency of cardiovascular disease. Micronutrients that can act as antioxidants-ascorbic acid (Vitamin C), a-tocopherol (Vitamin E), and co-enzyme Q10 have all been shown to prevent LDL oxidation in vitro and retard the progression of atherosclerosis in animal models.19, 20, 21, 22
In short, plasma levels of ox-LDL are a sensitive biomarker of atherosclerosis. Additionally, elevated MPO levels may be also measured to ascertain the degree of vascular damage.23
Elevated levels of ox-LDL are associated with accelerated atherogenesis, coronary artery disease, acute myocardial infarction, and stable and unstable angina. Elevated ox-LDL has also been associated with metabolic syndrome, impaired glucose tolerance/insulin resistance, and untreated overt hypothyroidism. Unfortunately, oxidized LDL nor MPO are rarely ordered or even discussed by primary care physicians.
The idea that low-density lipoproteins (LDL) are “bad,” is a gross oversimplification. The size of LDL particles and assessment of the number of LDL particles (LDL-Num) has been suggested as a more reliable method of assessing the potential of atherogenicity. There are basically two types of LDL in relationship to particle size: small dense LDL and large buoyant LDL. Small dense LDL particles are like little darts that tear holes in the lining of blood vessels, but large buoyant LDL particles are like large fluffy balls. They are unable to cause any damage and may actually prevent the small dense LDL from causing damage. For this reason, a simplistic cholesterol test that does not separate these two types of LDL cholesterol cannot adequately predict risk. The ratio of total-to-HDL cholesterol as well as non-HDL cholesterol, which is similar, are better predictors than total cholesterol or LDL cholesterol of the potential of atherosclerotic plaquing, but they are nowhere near as predictive as some of the newer markers, such as an LDL particle number.24, 25, 26
Lipoprotein (a) and C-Reactive Protein hs-CRP
Lipoprotein (a) discovered in the 1960s, has received little attention from researchers for the last decade due to the lack of understanding of its role in atherosclerosis. It is now known that Lp(a) exerts a strong affinity for binding to the blood vessel wall and can play a key role in developing atherosclerosis as a “partner in crime” with oxidized LDL.27, 28, 29 Both are known to be highly pro-inflammatory.
The risk attributable to Lp(a) may be due to its ability to bind and travel with oxidized LDL, attaching these toxic particles to the vessel walls, where they then may induce inflammation that can lead to blocked arteries. Oxidized LDL particles are strongly attached to Lp(a), this might explain why Lp(a) may be atherogenic.
C-reactive Protein (hs-CRP)
C-reactive protein (hs-CRP) is an acute-phase protein and its concentration in serum reflects the inflammatory condition of the patient. Thus, hs-CRP has become a commonly conducted test of systemic inflammation. It is also a better predictor of cardiovascular events than a simple LDL-cholesterol test. Whereas those with the highest level of LDL-cholesterol have a 1.5 increased risk factor of heart attack compared with those with the lowest levels, those with the highest level of hs-CRP have an increased risk factor of 2.3 compared with the lowest.30
Thus, individuals with elevated levels of LDL-cholesterol but low levels of hs-CRP have a lower risk of a heart attack than a person with low levels of LDL-cholesterol but high levels of hs-CRP. The primary reason LDL-cholesterol correlates with cardiovascular disease is that it is easily oxidized. Patients suffering from a heart attack have ox-LDL twice as high as patients with angina but no heart attack and 4 × as high as healthy controls.31
With most people exhibiting elevated LDL, some of it is likely to be the oxidized LDL cholesterol form, hence the apparent correlation of heart disease with elevated cholesterol. The point is that most doctors are only measuring total, LDL, and HDL cholesterol, however, what should really be measured clinically is oxidized LDL (ox-LDL), LDL particle size, lipoprotein(a), triglycerides, and c-reactive protein. These give us a much better idea of overall cardiovascular risk. It is also worth conducting HDL particle size as well. Research shows that individuals with the smallest HDL particles had the most unfavorable cardiometabolic risk profile whereas those with the largest HDL particles had the most favorable risk profile.32
The Intestinal Microbiome and Cholesterol
The intestine is home to trillions of microorganisms that include bacteria, fungi, parasites, and viruses. This “microbiota” is a hidden organ of the human system and regulates homeostasis or disease in an individual. In a healthy state, these interactions are symbiotic and influence a host’s nutrition, metabolism, energy, and immunity.33 Modern methodologies have given insights into the role of the gut in many processes such as the absorption, distribution, and extraction of nutrients, synthesis of vitamins, immunomodulation, and protection against pathogens.34
The composition of the microbiota can vary between people of the same ethnicity, age, or lifestyle because there is a complex interaction between classic genetic, diet, and environmental factors. There are over fifty bacterial phyla in the gut, yet there are two predominant phyla governing the gut microbiome: the Gram-positive Firmicutes (e.g., Enterococcus and Lactobacillus) and the Gram-negative Bacteroidetes (e.g., Bacteroides). The other phyla are present in variable numbers and include Actinobacteria (Bifidobacterium), Proteobacteria, and Verrucomicrobia.35
Overall, numerous factors such as the physiological conditions of the host (e.g., age and stress), dietary habits, environmental factors (e.g., use of medications especially antibiotic therapy), and intestinal infections can alter the diversity and composition in intestinal microorganisms creating a state of imbalance or “dysbiosis”. This state has been associated with the pathogenesis of both intestinal and extraintestinal disorders such as irritable bowel syndrome, metabolic syndrome, obesity, diabetes, and cardiovascular system disorders.36
Many findings suggest that the gut microbiota can alter blood lipid composition in particular cholesterol, through their role in bile acid metabolism and the generation of microbial products.37, 38, 39, 40
The use of probiotics and prebiotics has gained considerable popularity as these supplements help to establish a healthy intestinal microbiome. Specifically, probiotics introduce additional external microorganisms in the intestinal tract while prebiotics stimulate the growth rate of one or more of these microorganisms in the host. Thus, the possibility to modulate lipid profiles by targeting microbial communities with dietary interventions is a useful therapeutic tool to improve human health and improve cholesterol management.
Dietary Considerations
Simple changes in the diet can reduce cardiovascular risk by managing vascular endothelial dysfunction and achieve the same effect (if not better and safer) as problematic cholesterol-lowering drugs without also cutting off the body’s vital supply of cholesterol. Consumption of fruits and vegetables has been shown to protect vascular health by a variety of mechanisms driven by a wide array of plant nutrients (e.g., fiber, vitamins, carotenoids, alkaloids, nitrates, polyphenols, etc.).41
This protective relationship has been established for polyphenol intake, particularly compounds known as flavonoids, stilbenes, and tannins.42, 43, 44 Most of these compounds have been shown to exhibit antioxidant and anti-inflammatory activities to help reduce LDL oxidation and cellular injury, as well as compound-specific activities that trigger cellular processes that improve the actions of endothelial nitric oxide synthase.45, 46
A summary of all the fruits, vegetables, and phytonutrients that have beneficial effects on vascular endothelial function is lengthy and beyond the scope of this article. Most research has focused on ingredients that are common in the diet, or that are readily available as dietary supplement ingredients such as blueberries, pomegranate, cranberries, hawthorn (leaf and berries), olives., green tea, resveratrol, quercetin, curcuminoids, anthocyanidins, berberine, etc. The following are additional suggestions:
Avoid hydrogenated and partially hydrogenated oils, trans-fats, and polyunsaturated industrial seed oils such as corn, sunflower, cotton seed, peanut, soy, and canola oil. These are all linked to cellular congestion and inflammation, which can lead to chronic diseases like heart disease.
Avoid refined sugars, especially fructose, known to stimulate clumping of the blood platelets. Refined sugars are an anti-nutrient and offer insignificant amounts of vitamins and minerals, but simultaneously rob the body of nutrient stores. This can lead to chronic diseases such as heart disease. High glycemic or refined sugar cause elevated glucose levels resulting in insulin spikes that can lead to premature aging and degenerative diseases such as heart disease, diabetes, and cancer.
Consider supplementing cod liver oil as it is an excellent dietary source of anti-inflammatory vitamin A, vitamin D, and EPAs
Consider supplementing with evening primrose, borage, or black currant oil, all sources of Gamma linolenic acid (GLA). GLA is also present in trace amounts in green leafy vegetables and nuts. The most significant source of GLA for infants is breast milk. GLA creates anti-inflammatory prostaglandins.
Eat foods that contain abundant trace minerals, particularly copper; copper deficiency is associated with clot formation and inflammation in the arteries. Plant sources include spirulina, shiitake mushrooms, leafy greens like spinach, kale, and Swiss chard, and the following fruits: avocados, guavas, pomegranate, blackberries, kiwifruit, mangos, and pineapple.
Choose better quality meat, if eating meat. Hundreds of studies link commercial meat (i.e., industrial farm lot meat) to heart disease. This could be due to grain (many of them -particularly corn- now genetically modified) being fed to these animals, who are historically grass eaters. This sub-standard cattle diet changes the fatty acid ratios in the meat, denaturing good fats and making the meat more inflammatory. This leads to chronic diseases like heart disease. Also, these factory-farmed cattle are typically pumped full of hormones and antibiotics. The inflammatory toxins accumulate in the fat. Choose the leanest meat possible, to avoid the accumulation of toxins and other inflammatory properties in the animal fat.
Avoid reduced-fat milk and powdered milk products (such as powdered whey); they contain oxidized cholesterol, shown to cause endothelial dysfunction of the artery wall.
Some natural antioxidants, such as Vitamin C, alpha- and gamma-tocopherol, rosemary oleoresin extract, Coenzyme Q10, and quercetin inhibit the oxidation of cholesterol.
Consider supplementing pre-and probiotics – rotate culture over time.
Additionally, do not undervalue the role that stress and adequate sleep play in maintaining the healthy metabolic pathways the body utilizes to promote normal cholesterol levels in the body.
It is also thorough to ensure the liver, thyroid, insulin, and general level of inflammation are all normal as well. The following laboratory tests can better determine cardiovascular risk status.
Total cholesterol to HDL ratio (this assesses the amount of HDL available to recycle LDL from the blood before it has a chance to become oxidized)
LDL particle size via an NMR or VAP test (or an APOB test) which evaluates particle size and assesses oxidation-prone state (this is by far more diagnostic than just an LDL value alone)
Oxidized LDL level
Lp(a)
Hs-CRP
Thyroid function – TSH, Free T4, Free T3
Insulin function – fasting Insulin Level
Hemoglobin A1C
References:
1. Andrews, Norma W., Patricia E. Almeida, and Matthias Corrotte. "Damage control: cellular mechanisms of plasma membrane repair." Trends in cell biology 24, no. 12 (2014): 734-742.
2. Lee, Anthony G. "How lipids affect the activities of integral membrane proteins." Biochimica et Biophysica Acta (BBA)-Biomembranes 1666, no. 1-2 (2004): 62-87.
3. Hussain, Ghulam, Jing Wang, Azhar Rasul, Haseeb Anwar, Ali Imran, Muhammad Qasim, Shamaila Zafar et al. "Role of cholesterol and sphingolipids in brain development and neurological diseases." Lipids in health and disease 18, no. 1 (2019): 1-12.
4. Lecis, C., and M. Segatto. "Cholesterol homeostasis imbalance and brain functioning: neurological disorders and behavioral consequences." J. Neurol. Neurol. Disord 1, no. 1 (2014): 101.
5. Hu, Jie, Zhonghua Zhang, Wen-Jun Shen, and Salman Azhar. "Cellular cholesterol delivery, intracellular processing and utilization for biosynthesis of steroid hormones." Nutrition & metabolism 7, no. 1 (2010): 1-25.
6. Payne, Anita H., and Dale B. Hales. "Overview of steroidogenic enzymes in the pathway from cholesterol to active steroid hormones." Endocrine reviews 25, no. 6 (2004): 947-970.
7. Norlin, Maria, and Kjell Wikvall. "Enzymes in the conversion of cholesterol into bile acids." Current molecular medicine 7, no. 2 (2007): 199-218.
8. Tall, Alan R., and Laurent Yvan-Charvet. "Cholesterol, inflammation and innate immunity." Nature Reviews Immunology 15, no. 2 (2015): 104-116.
9. King, Ryan J., Pankaj K. Singh, and Kamiya Mehla. "The cholesterol pathway: impact on immunity and cancer." Trends in immunology 43, no. 1 (2022): 78-92.
10. Fessler, Michael B. "The intracellular cholesterol landscape: dynamic integrator of the immune response." Trends in immunology 37, no. 12 (2016): 819-830.
11. Kita, Toru, Noriaki Kume, Manabu Minami, Kazutaka Hayashida, Toshinori Murayama, Hideto Sano, Hideaki Moriwaki et al. "Role of oxidized LDL in atherosclerosis." Annals of the New York Academy of Sciences 947, no. 1 (2001): 199-206.
12. YlÄ‐Herttuala, Seppo. "Oxidized LDL and Atherogenesis a." Annals of the New York Academy of Sciences 874, no. 1 (1999): 134-137.
13. Mertens, A. N. N., and Paul Holvoet. "Oxidized LDL and HDL: antagonists in atherothrombosis." The FASEB journal 15, no. 12 (2001): 2073-2084.
14. Ghandehari H, Kamal-Bahl S, Wong ND. Prevalence and extent of dyslipidemia and recommended lipid levels in US adults with and without cardiovascular comorbidities: the National Health and Nutrition Examination Survey 2003–2004. Am Heart J. 2008;156(1):112–119. [PubMed] [Google Scholar]
15. Staprans, Ilona, Xian‐Mang Pan, Joseph H. Rapp, and Kenneth R. Feingold. "The role of dietary oxidized cholesterol and oxidized fatty acids in the development of atherosclerosis." Molecular nutrition & food research 49, no. 11 (2005): 1075-1082.
16. Valenzuela A, Sanhueza J, Nieto S. Cholesterol oxidation: health hazard and the role of antioxidants in prevention. Biol Res. 2003;36:291–302.
17. Smith, Leland L. "Another cholesterol hypothesis: cholesterol as antioxidant." Free Radical Biology and Medicine 11, no. 1 (1991): 47-61.
18. Leonarduzzi, Gabriella, Barbara Sottero, and Giuseppe Poli. "Oxidized products of cholesterol: dietary and metabolic origin, and proatherosclerotic effects." The Journal of nutritional biochemistry 13, no. 12 (2002): 700-710.
19. Niki, Etsuo, and Noriko Noguchi. "Effects of antioxidants against atherosclerosis." Oxygen/Nitrogen Radicals: Cell Injury and Disease (2002): 19-25.
20. Aviram, M. "Review of human studies on oxidative damage and antioxidant protection related to cardiovascular diseases." Free Radical Research 33 (2000): S85-97.
21. Carew, T. E., Schwenke, D. C., & Steinberg, D. (1987). Antiatherogenic effect of probucol unrelated to its hypocholesterolemic effect: evidence that antioxidants in vivo can selectively inhibit low density lipoprotein degradation in macrophage-rich fatty streaks and slow the progression of atherosclerosis in the Watanabe heritable hyperlipidemic rabbit. Proceedings of the National Academy of Sciences, 84(21), 7725-7729.
22. Steinberg, Daniel. "Role of oxidized LDL and antioxidants in atherosclerosis." Nutrition and biotechnology in heart disease and cancer (1995): 39-48.
23. Heslop, Claire L., Jiri J. Frohlich, and John S. Hill. "Myeloperoxidase and C-reactive protein have combined utility for long-term prediction of cardiovascular mortality after coronary angiography." Journal of the American College of Cardiology 55, no. 11 (2010): 1102-1109.
24. van der Steeg, Wim A., Ingar Holme, S. Matthijs Boekholdt, Mogens Lytken Larsen, Christina Lindahl, Erik SG Stroes, Matti J. Tikkanen et al. "High-density lipoprotein cholesterol, high-density lipoprotein particle size, and apolipoprotein AI: significance for cardiovascular risk: the IDEAL and EPIC-Norfolk studies." Journal of the American College of Cardiology 51, no. 6 (2008): 634-642.
25. Mackey, Rachel H., Philip Greenland, David C. Goff, Donald Lloyd-Jones, Christopher T. Sibley, and Samia Mora. "High-density lipoprotein cholesterol and particle concentrations, carotid atherosclerosis, and coronary events: MESA (multi-ethnic study of atherosclerosis)." Journal of the American College of Cardiology 60, no. 6 (2012): 508-516.
26. El Harchaoui, Karim, Wim A. van der Steeg, Erik SG Stroes, Jan Albert Kuivenhoven, James D. Otvos, Nicholas J. Wareham, Barbara A. Hutten, John JP Kastelein, Kay-Tee Khaw, and S. Matthijs Boekholdt. "Value of low-density lipoprotein particle number and size as predictors of coronary artery disease in apparently healthy men and women: the EPIC-Norfolk Prospective Population Study." Journal of the American College of Cardiology 49, no. 5 (2007): 547-553.
27. Kamstrup, Pia R. "Lipoprotein (a) and cardiovascular disease." Clinical chemistry 67, no. 1 (2021): 154-166.
28. Miksenas, Hannah, James L. Januzzi, and Pradeep Natarajan. "Lipoprotein (a) and cardiovascular diseases." JAMA 326, no. 4 (2021): 352-353.
29. Malaguarnera, Michele, Marco Vacante, Cristina Russo, Giulia Malaguarnera, Tijana Antic, Lucia Malaguarnera, Rita Bella, Giovanni Pennisi, Fabio Galvano, and Alessandro Frigiola. "Lipoprotein (a) in cardiovascular diseases." BioMed research international 2013 (2013).
30. Ridker, Paul M., Nader Rifai, Lynda Rose, Julie E. Buring, and Nancy R. Cook. "Comparison of C-reactive protein and low-density lipoprotein cholesterol levels in the prediction of first cardiovascular events." New England journal of medicine 347, no. 20 (2002): 1557-1565.
31. Ehara S, Ueda M, Naruko T, et al. Elevated levels of oxidized low density lipoprotein show a positive relationship with the severity of acute coronary syndromes. Circulation. 2001;103(15):1955–1960.
32. Arsenault, Benoit J., Isabelle Lemieux, Jean-Pierre Després, Pascale Gagnon, Nicholas J. Wareham, Erik SG Stroes, John JP Kastelein, Kay-Tee Khaw, and S. Matthijs Boekholdt. "HDL particle size and the risk of coronary heart disease in apparently healthy men and women: the EPIC-Norfolk prospective population study." Atherosclerosis 206, no. 1 (2009): 276-281.
33. Malard, Florent, Joel Dore, Béatrice Gaugler, and Mohamad Mohty. "Introduction to host microbiome symbiosis in health and disease." Mucosal Immunology 14, no. 3 (2021): 547-554.
34. Jandhyala, Sai Manasa, Rupjyoti Talukdar, Chivkula Subramanyam, Harish Vuyyuru, Mitnala Sasikala, and D. Nageshwar Reddy. "Role of the normal gut microbiota." World journal of gastroenterology: WJG 21, no. 29 (2015): 8787.
35. Mishra, Alok Kumar, Vinay Dubey, and Asit Ranjan Ghosh. "Obesity: An overview of possible role (s) of gut hormones, lipid sensing and gut microbiota." Metabolism 65, no. 1 (2016): 48-65.
36. Kriaa, Aicha, Mélanie Bourgin, Aline Potiron, Héla Mkaouar, Amin Jablaoui, Philippe Gérard, Emmanuelle Maguin, and Moez Rhimi. "Microbial impact on cholesterol and bile acid metabolism: current status and future prospects." Journal of lipid research 60, no. 2 (2019): 323-332.
37. Kriaa, Aicha, Mélanie Bourgin, Aline Potiron, Héla Mkaouar, Amin Jablaoui, Philippe Gérard, Emmanuelle Maguin, and Moez Rhimi. "Microbial impact on cholesterol and bile acid metabolism: current status and future prospects." Journal of lipid research 60, no. 2 (2019): 323-332.
38. Rabot, Sylvie, Mathieu Membrez, Aurélia Bruneau, Philippe Gérard, Taoufiq Harach, Mireille Moser, Frederic Raymond, Robert Mansourian, and Chieh J. Chou. "Germ‐free C57BL/6J mice are resistant to high‐fat‐diet‐induced insulin resistance and have altered cholesterol metabolism." The FASEB Journal 24, no. 12 (2010): 4948-4959.
39. Le Roy, Tiphaine, Emelyne Lécuyer, Benoit Chassaing, Moez Rhimi, Marie Lhomme, Samira Boudebbouze, Farid Ichou et al. "The intestinal microbiota regulates host cholesterol homeostasis." BMC biology 17, no. 1 (2019): 1-18.
40. Schoeler, Marc, and Robert Caesar. "Dietary lipids, gut microbiota and lipid metabolism." Reviews in endocrine and metabolic disorders 20 (2019): 461-472.
41. Kamaruddin, Nur Aqilah, Muhammad Nazrul Hakim Abdullah, Jun Jie Tan, Vuanghao Lim, Lai Yen Fong, Siti Aisyah Abd Ghafar, and Yoke Keong Yong. "Vascular Protective Effect and Its Possible Mechanism of Action on Selected Active Phytocompounds: A Review." Evidence-Based Complementary and Alternative Medicine 2022 (2022).
42. Yamagata, Kazuo, Motoki Tagami, and Yukio Yamori. "Dietary polyphenols regulate endothelial function and prevent cardiovascular disease." Nutrition 31, no. 1 (2015): 28-37.
43. Grosso, Giuseppe, Justyna Godos, Walter Currenti, Agnieszka Micek, Luca Falzone, Massimo Libra, Francesca Giampieri et al. "The effect of dietary polyphenols on vascular health and hypertension: Current evidence and mechanisms of action." Nutrients 14, no. 3 (2022): 545.
44. Stoclet, Jean-Claude, Thierry Chataigneau, Mamadou Ndiaye, Min-Ho Oak, Jasser El Bedoui, Marta Chataigneau, and Valérie B. Schini-Kerth. "Vascular protection by dietary polyphenols." European journal of pharmacology 500, no. 1-3 (2004): 299-313.
45. Serreli, Gabriele, and Monica Deiana. "Role of Dietary Polyphenols in the Activity and Expression of Nitric Oxide Synthases: A Review." Antioxidants 12, no. 1 (2023): 147.
46. Ahmadi, Ali, Tannaz Jamialahmadi, and Amirhossein Sahebkar. "Polyphenols and atherosclerosis: A critical review of clinical effects on LDL oxidation." Pharmacological Research (2022): 106414.